In a new interview on AZoQuantum, we spoke to theoretical physicist Markus Müller about his research exploring a novel qubit design methodology. The team also discovered that rare-earth ions could be used to create highly coherent qubits in a dense system. In the following piece, we discuss these findings and more.
Please can you introduce yourself and your professional background?
I am a theoretical physicist working on a broad range of topics between quantum condensed matter and the statistical physics of disordered systems. I received my Ph.D. at Orsay, Paris. After two postdocs in the USA, I joined the International Center of Theoretical Physics in Trieste in 2009, where I established myself as an expert on many-body localization, quantum glasses, quantum transport, and out-of-equilibrium physics.
In 2015, I moved to the Paul Scherrer Institute in Switzerland, where one of my main interests is the manifold magnetic incarnations of the interplay between disorder, interactions, and quantum fluctuations.
Can you describe the traditional approach to qubit design and the limitations that led your team to explore a new method?
Traditionally, qubits are fabricated in what is hoped to be deterministic processes, generating and placing the relevant structures (e.g., for Josephson devices) one by one. This may, however, not always be possible or efficient.
In the context of magnetic impurities, whose potential as quantum coherent objects we wanted to explore, techniques for targeted implantation of individual magnetic ions are not much developed yet, and those that exist are very laborious and have strong limits to their spatial precision.
What initially prompted the research team to consider using a cluttered environment for qubits instead of pursuing ultra-clean materials?
The aim was to demonstrate the possibility of creating coherent superpositions of crystal field excitations of rare earth ions, as that capability is a crucial ingredient in the quantum computing scheme we proposed earlier [PRX Quantum 2, 010213 (2021)].
For such an application, one is interested in a good route to scale up as well as the possibility of strong interactions between excited qubits, that is, a relatively high density of qubits. The same is true when using qubits as sensors or bulk properties of the material or simply when aiming at quantum control of a magnetic material.
The high density has the further advantage that the coherence of the ensemble can be probed collectively using a relatively simple Hahn echo, producing an appreciable signal above the noise level.
How did your team come to the realization that rare-earth ions could be used to create highly coherent qubits in a dense system?
The fact that rare islands of high coherence emerge in a rather densely doped system was an unanticipated discovery. We noted very sharp peaks in the echo signal that stemmed from coherent pairs.
Can you elaborate on the process of identifying and utilizing terbium pairs within yttrium lithium fluoride crystals?
We detect the presence of pairs in the material by their signal in our Hahn echo experiment.
However, we do not attempt to locate individual pairs in the material. We use the pair echo and its temporal decay to infer properties of the dynamics among the remaining terbium atoms. Those form an ensemble of dipolar coupled, disordered two-level systems whose many-body dynamics are not fully understood.
By using the pairs as sensors, we were able to establish that the different states of terbium atoms have vastly different lifetimes and, thus, energy diffusion constants several orders of magnitude lower than a dimensional estimate based on dipolar interactions would suggest.
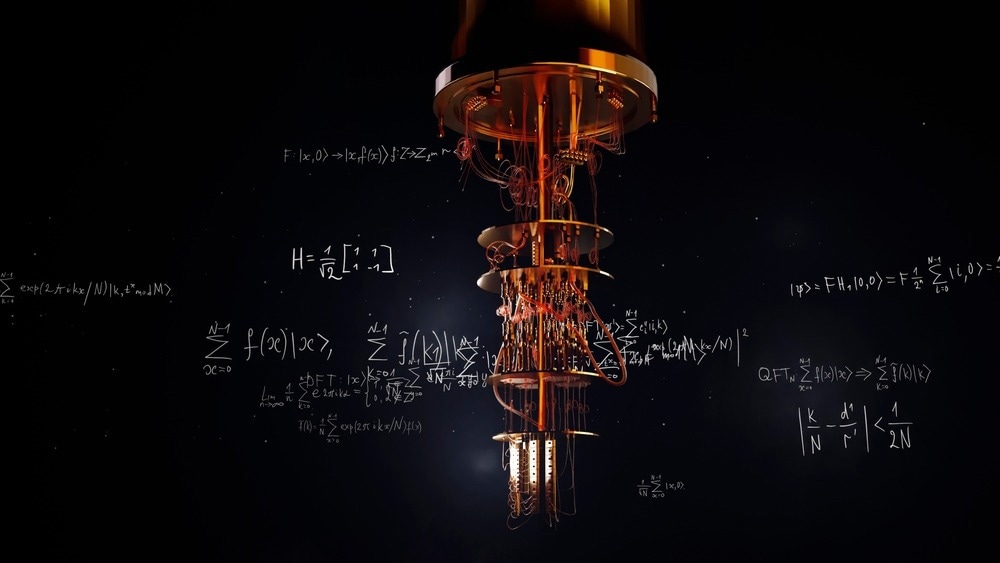
Image Credit: Lia Koltyrina/Shutterstock.com
This can be seen as a manifestation of (quasi-)localization, a requirement for a useful, independent qubit. Future experiments in nuclear spin-free samples may shed yet more light on the mechanism behind this diffusion and its dependence on concentration and disorder level.
What were the key challenges you faced in proving that these qubit pairs could maintain longer coherences?
The experimental challenge was to operate at relatively unusual microwave frequencies and to design an appropriate resonator. The fact that the pairs are more coherent is directly apparent from the much slower decay of the Hahn echo with time.
However, to extract useful information from that decay, one has to disentangle the various sources that contribute to the degrading of the echo. The latter was a major challenge, as it required the simultaneous fitting of echo data at different concentrations for many frequencies and under various decoupling schemes.
How does the interaction between the qubits and their environment in this new approach differ from traditional methods?
Most dephasing channels (diffusion of excitations, dephasing by nuclear spins) are essentially the same as in other situations. However, the dephasing we were interested in, namely the one induced by the dynamics of other terbium ions that we wanted to probe, occurs through a non-standard channel.
The external field brings the pair qubits to have vanishing magnetic moments. Thus, they essentially do not sense the fluctuating magnetic fields when other terbium flip. What they do sense, however, is the fluctuation in the quantum mechanical energy shift, which stems from virtual spin flips with a neighbor.
The latter depends on the spin state of the neighbors and changes when the neighbors undergo dynamics. We refer to this unusual type of dephasing interaction as ring-exchange.
What does this discovery mean for the future scalability of quantum computing technologies?
Our discovery suggests that it can be a viable approach to generate a large set of potential qubits in a stochastic rather than deterministic manner and to post-select the highly coherent qubits.
While this might become an element in the design for quantum computation (where individual addressability, read-out, and entangling gates remain big challenges to address), we see its primary use predominantly in the realm of sensing.
What are the immediate next steps in your research?
(i) To optimize the sensitivity of the pair quantum sensors, we will repeat the creation of quantum superposition of local electro(-nuclear) states in host materials that are free of nuclear spins (so as to minimize unwanted sources of magnetic noise, which in LiYF4 arises from the host's fluorine spins). A systematic study in terms of dopant concentration is envisioned to probe the crossover in the dipolar diffusion.
(ii) We will try to achieve similar coherent superpositions of higher-lying ion states, extending the range of excitation frequencies from the microwaves (30 GHz) we use now to the optical range where the availability of strong lasers allows for faster excitation times (Rabi frequencies). Promising preliminary results in this direction have already been obtained.
(iii) We are exploring the use of pairs of dopants, also in the context of quantum information processing/computing with dopants in semiconductors.
More Interviews on AZoQuantum: Modified Newtonian Dynamics and the Search for a Ninth Planet
About Markus Müller
I am a condensed matter theorist at Switzerland’s National Lab, the Paul Scherrer Institute, working closely with various experimental groups in the Laboratory for X-ray Nanoscience and Technologies and in the context of this project, in particular with the Quantum Photon Science Group (www.psi.ch/en/lxn/quantum-technologies), respectively the Quantum Technologies group at the Department of Physics and the Quantum Center of ETH Zurich (www.qt.ethz.ch).
As members of the Quantum Technology Collaboration at our institute, we are trying to leverage phenomena and effects from quantum condensed matter to applications in quantum technology (www.psi.ch/en/quantum-technologies-collaboration), using our unique large-scale facilities, such as the Swiss Light Source synchrotron, the SwissFEL X-ray free-electron laser or the ETHZ-PSI Quantum Computing Hub.
Disclaimer: The views expressed here are those of the interviewee and do not necessarily represent the views of AZoM.com Limited (T/A) AZoNetwork, the owner and operator of this website. This disclaimer forms part of the Terms and Conditions of use of this website.