A team of scientists have developed a new imaging technique for observing hydrodynamic electron flow in three-dimensional materials that is shifting the long-held assumptions of this activity. New research has revealed that hydrodynamic electron flow in three-dimensional conductors occurs more similarly to the movement of gas, rather than a fluid, as was previously theorized.
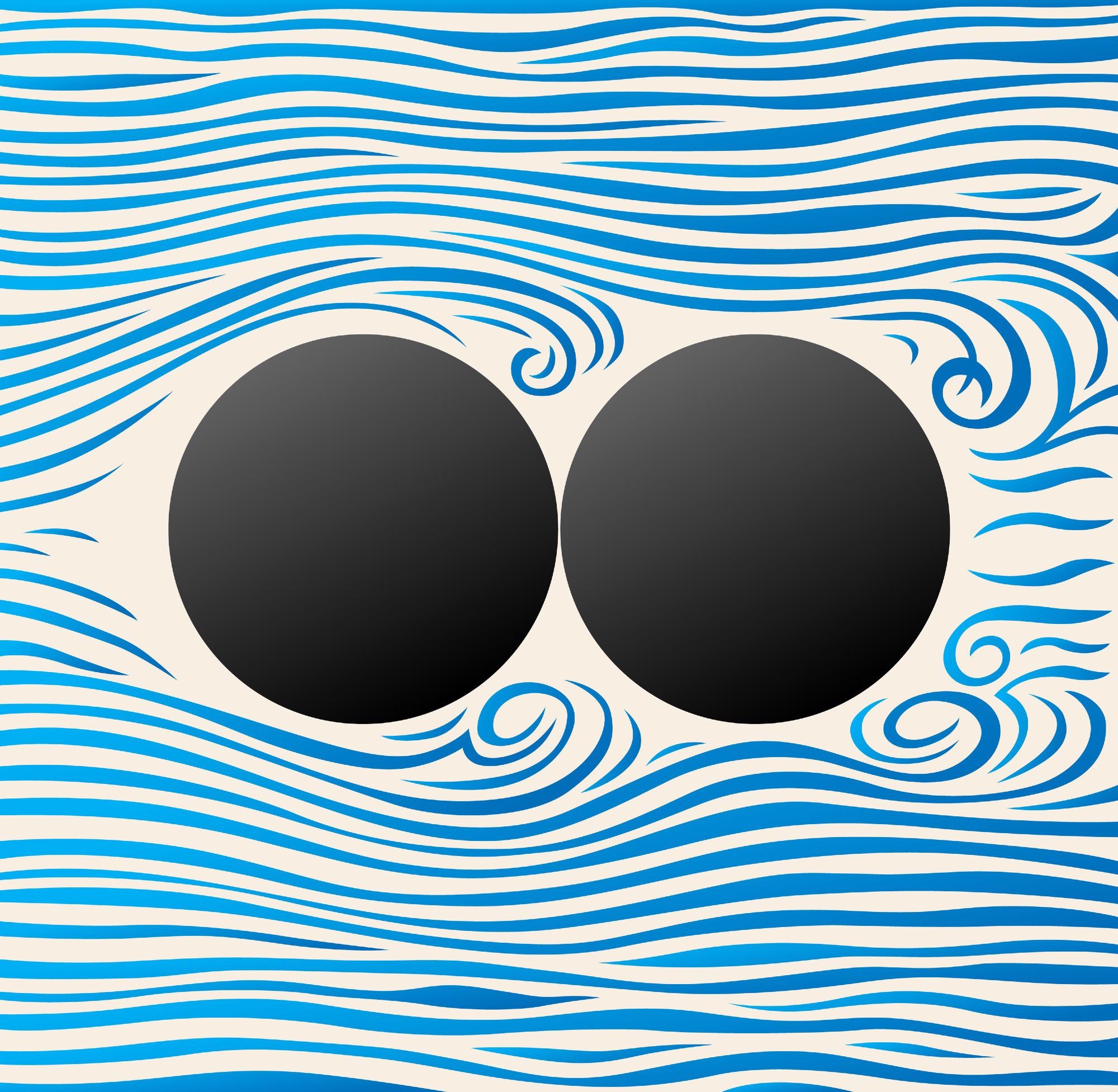
Image Credit: Dn Br/Shutterstock.com
This new data opens up new avenues for exploring hydrodynamic flow and prominent electron interactions, which will likely be leveraged in a number of new applications.
Shifting Perceptions of the Hydrodynamics of Electrons Moving Through Materials
For many years, physicists theorized that electrons flowed through materials like a fluid. However, recent advances in technology that have allowed scientists to observe these effects much more closely in two-dimensional materials have shifted this perception.
Now, scientists hypothesize that electrons actually move through materials in a gas-like manner, rather than a fluid-like manner, as the particles do not interact with one another.
Professor of Physics and of Applied Physics at the Harvard John A. Paulson School of Engineering and Applied Sciences (SEAS), Amir Yacoby, Professor of Physics and Professor Applied Physics at Harvard, Philip Kim, and Ronald Walsworth who at the time was with the Department of Physics at Harvard, were those who were amongst the very first to obtain imagery of electrons flowing through graphene like a liquid.
The images, published in 2020, provided a new and exciting avenue for physicists to explore and deepen their knowledge of how electrons interact, thus providing clues to how electrons may be controlled. The only limitation of this groundbreaking research was that it only visualized the movement of electrons through two-dimensional materials.
This is significant because the hydrodynamics of electrons moving through three-dimensional materials had remained elusive until new research was published this year in the journal Nature Physics.
Electrons Limit Their Interactions When Densely Packed Into a Material
Scientists from Harvard and MIT teamed up to investigate the nature of hydrodynamic electron flow in three-dimensional materials, which they observed for the first time via the development of a novel imaging technique.
The hydrodynamic flow of electrons hinges on the strong interactions between electrons, in a similar way to which fluids like water rely on strong particle-to-particle interactions. Research has shown that higher densities of electrons within a lateral flow result in weaker interactions.
To achieve efficient flow, electrons in high density materials form themselves into arrangements that limit intra-electron interactions. While it seems as though the opposite should be true–that the more densely packed the electrons are, the more likely they are to interact–they move into arrangements that prevent this in order to maintain efficient movement.
Previously, the only work that had given inferences about hydrodynamic effects was mainly deduced from transport measurements. The new study gives a more accurate view of these effects via the use of new quantum probes of electron correlations.
A novel cryogenic scanning probe
The US-based researchers established a novel cryogenic scanning probe that leverages the nitrogen-vacancy defect in diamonds, to observe the flow of electrons in a three-dimensional material. The team used a quantum sensor sensitive enough to detect minute changes within the local magnetic field of a current flow through layered semimetal tungsten ditelluride to visualize the magnetic structure of the material.
With this method, the team found evidence of a hydrodynamic flow within the material. In addition, they were also able to demonstrate that the hydrodynamic character of the current is highly dependent on the temperature.
According to the study, hydrodynamic flow occurs within a small window where the temperature is just right—not too hot and not too cold. The method allowed researchers to scan across an extensive temperature range, which was vital to observing the effect.
It was proposed that the electrons within high density materials interact via quantum vibrations of the atomic lattice, rather than through direct interactions. These quantum vibrations are known as phonons.
Conclusion
The new research opens up an exciting avenue to be explored which will be fundamental to uncovering more about the hydrodynamic flow and prominent electron interactions in high-carrier-density materials.
The new technique established by the team in the current research gives scientists the ability to image and engineer such hydrodynamic flows in three-dimensional materials as a function of temperature, making it possible to work towards near dissipation-less electronics in nanoscale devices.
In addition, it will also provide vital insights into electron–electron interactions that will likely be leveraged into numerous applications. Finally, the research also opens up the opportunity to investigate non-classical fluid behavior in hydrodynamic electron flow—steady-state vortices for example.
It is likely that we will see much more research conducted in this field in the coming years.
References and Further Reading:
Science Daily. (2021). The first glimpse of hydrodynamic electron flow in 3D materials. [Online]. Available at: https://www.sciencedaily.com/releases/2021/09/210916142826.htm (Accessed 21 October 2021)
Gooth, J., Menges, F., Kumar, N., Süβ, V., Shekhar, C., Sun, Y., Drechsler, U., Zierold, R., Felser, C. and Gotsmann, B., (2018). Thermal and electrical signatures of a hydrodynamic electron fluid in tungsten diphosphide. Nature Communications, 9(1). https://www.nature.com/articles/s41467-018-06688-y#citeas
Vool, U., Hamo, A., Varnavides, G., Wang, Y., Zhou, T., Kumar, N., Dovzhenko, Y., Qiu, Z., Garcia, C., Pierce, A., Gooth, J., Anikeeva, P., Felser, C., Narang, P. and Yacoby, A., (2021). Imaging phonon-mediated hydrodynamic flow in WTe2. Nature Physics,. https://www.nature.com/articles/s41567-021-01341-w#citeas
Disclaimer: The views expressed here are those of the author expressed in their private capacity and do not necessarily represent the views of AZoM.com Limited T/A AZoNetwork the owner and operator of this website. This disclaimer forms part of the Terms and conditions of use of this website.