It is possible to place the majority of materials into one of two categories just by looking at their subatomic particles.
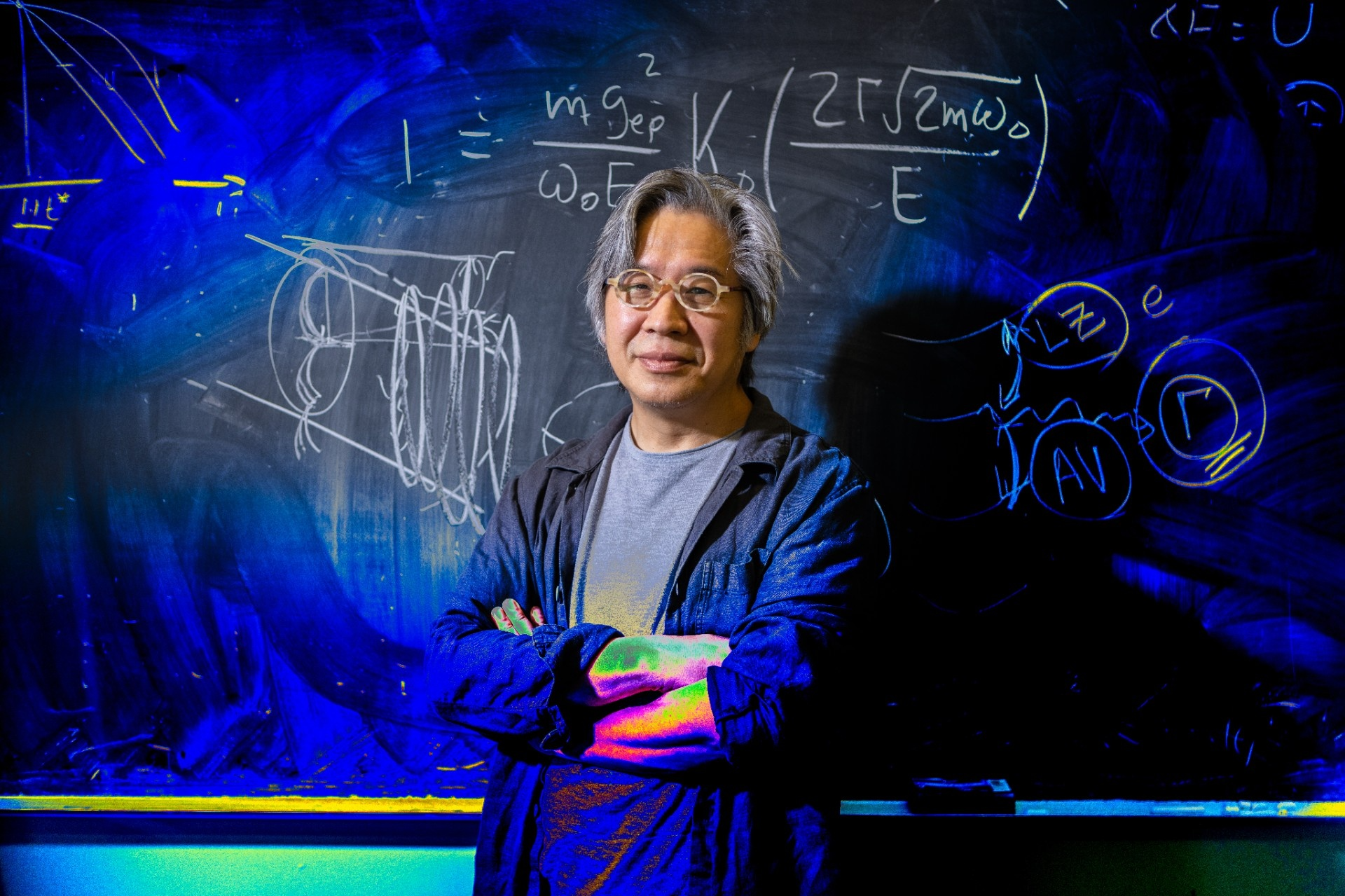
UB physics professor Jong Han is the lead author of a new study that helps solve a longstanding physics mystery on how insulators transition into metals via an electric field, a process known as resistive switching. Image Credit: Douglas Levere, University at Buffalo
Metals—like iron and copper—consist of free-flowing electrons that enable them to perform electricity, while insulators—like rubber and glass—keep their electrons bound tightly and hence do not conduct electricity.
Insulators have the potential to convert into metals while being subjected to an intense electric field. This provides tantalizing possibilities for supercomputing and microelectronics, but the physics present behind this phenomenon known as resistive switching is yet to be understood clearly.
Questions like how large an electric field is required are intensely debated by researchers.
I have been obsessed by that.
Jong Han, Condensed Matter Theorist, University at Buffalo
Han, Ph.D., professor of physics at the College of Arts and Sciences, is the lead author of a study that takes a new method to answer a persistent puzzle concerning insulator-to-metal transitions.
The study, entitled, “Correlated insulator collapse due to quantum avalanche via in-gap ladder states,” was published in the journal Nature Communications on May 2023.
Electrons Move Through Quantum Paths
According to Han, the variation between insulators and metals comes under quantum mechanical principles, which dictate that electrons are quantum particles, and their energy levels are available in bands that consist of forbidden gaps.
Since the 1930s, the Landau-Zener formula has been a blueprint for identifying the size of the electric field required to push an electron’s insulator from its lower bands to its upper bands. However, experiments performed in the decades since have displayed materials need a much smaller electric field—nearly 1,000 times smaller—compared to the Landau-Zener formula estimated.
So, there is a huge discrepancy, and we need to have a better theory.
Jong Han, Condensed Matter Theorist, University at Buffalo
For this issue to be resolved, Han decided to consider a different question: What takes place when electrons present already in the upper band of an insulator have been pushed?
A computer simulation of resistive switching was made by Han that accounted for the existence of electrons in the upper band. It displayed that a comparatively small electric field could activate a failure of the gap between the lower and upper bands, thereby making a quantum path for the electrons to go up and down between the bands.
To make an analogy, Han stated the following.
Imagine some electrons are moving on a second floor. When the floor is tilted by an electric field, electrons not only begin to move but previously forbidden quantum transitions open up and the very stability of the floor abruptly falls apart, making the electrons on different floors flow up and down.
Jong Han, Condensed Matter Theorist, University at Buffalo
Han stated, “Then, the question is no longer how the electrons on the bottom floor jump up, but the stability of higher floors under an electric field.”
Han states that this idea helps resolve a few of the differences in the Landau-Zener formula. Also, it offers few clarities to the debate over insulator-to-metal transitions that have been caused by electrons themselves or those by extreme heat. The simulation of Han denotes the quantum avalanche is not activated by heat.
But the complete insulator-to-metal transition does not take place until the separate temperatures of the phonons and electrons—quantum vibrations of the crystal’s atoms—equilibrate. This displays that the mechanisms for thermal and electronic switching are not exclusive of each other, states Han, but could rather arise concurrently.
Han stated, “So, we have found a way to understand some corner of this whole resistive switching phenomenon. But I think it's a good starting point.”
Research Could Improve Microelectronics
The study performed was co-authored by Jonathan Bird, Ph.D., professor and chair of electrical engineering in UB’s School of Engineering and Applied Sciences, who offered experimental context.
Bird’s team has been studying the electrical properties of emergent nanomaterials that displayed novel states at low temperatures, which could teach scientists a lot regarding the complicated physics that rules electrical behavior.
“While our studies are focused on resolving fundamental questions about the physics of new materials, the electrical phenomena that we reveal in these materials could ultimately provide the basis of new microelectronic technologies, such as compact memories for use in data-intensive applications like artificial intelligence,” stated Bird.
Also, the study could be vital for areas like neuromorphic computing, which tries to imitate the electrical stimulation of the human nervous system.
“Our focus, however, is primarily on understanding the fundamental phenomenology,” Bird says.
The other authors of the study include UB physics Ph.D. student Xi Chen; Ishiaka Mansaray, who received a Ph.D. in physics and is currently a postdoc at the National Institute of Standards and Technology; and Michael Randle, who received a Ph.D. in electrical engineering and is now a postdoc at the Riken research institute in Japan.
Other authors include international scientists representing École Normale Supérieure, French National Centre for Scientific Research (CNRS) in Paris; Pohang University of Science and Technology; and the Center for Theoretical Physics of Complex Systems, Institute for Basic Science.
Since publishing the paper, Han has engineered an analytic theory that corresponds to the computer’s calculation well. Yet, there is more for him to examine, like the exact conditions required for a quantum avalanche to occur.
Han stated, “Somebody, an experimentalist, is going to ask me, ‘Why didn’t I see that before? Some might have seen it, some might not have. We have a lot of work ahead of us to sort it out.”