New research has determined that the Sun’s powerful magnetic field influenced Mercury’s core, thus showing how stars exert control over the composition of their planets. Despite being one of the solar system’s smallest planets, Mercury has been found to possess a huge core with a high concentration of iron. The core of Mercury is believed to possess a liquid outer shell, just like Earth’s. But, unlike our planet, research indicates that this liquid shell surrounds a solid iron inner core for Mercury.
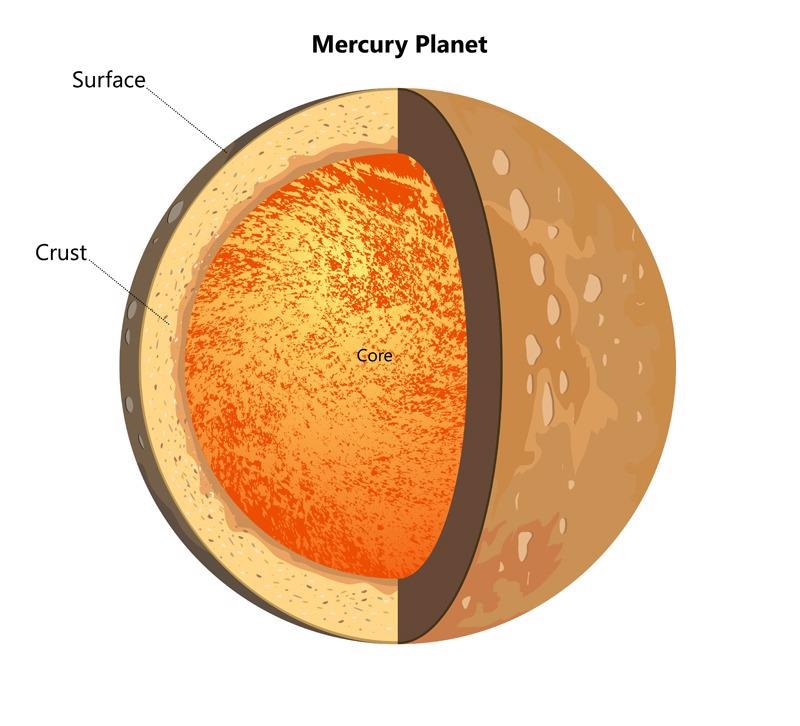
Image Credit: Shutterstock / mapichai
What makes this core so extraordinary is that it accounts for three-quarters of Mercury’s total mass. Compare that to the solar system’s other rocky bodies, Earth, Mars and Venus whose cores only account for around one-third of their total mass.
Until now, scientists had believed that Mercury possesses such a large core in comparison to its mantle — the layer that exists between a planet’s core and its crust — due to collisions with other bodies in the solar system. These impacts, which occurred during the solar system’s formation, were believed to have blown away the planet’s rocky mantle leaving behind a dense iron core. New research is challenging this idea, however.
In collaboration with Takashi Yoshizaki, Tohoku University, University of Maryland professor of geology William McDonough has devised a model of density, mass and iron content for rocky worlds, which indicates that proximity to a star’s powerful magnetic field could give rise to conditions like those found beneath the surface of Mercury.
The four inner planets of our solar system — Mercury, Venus, Earth and Mars — are made up of different proportions of metal and rock. There is a gradient in which the metal content in the core drops off as the planets get farther from the Sun.
William McDonough, Professor of Geology, University of Maryland
McDonough and Yoshizaki are the authors of a paper published in the journal Progress in Earth and Planetary Science that details the model, its development, and the impact it has on our understanding of Mercury’s interior.
“Our paper explains how this happened by showing that the distribution of raw materials in the early forming solar system was controlled by the Sun’s magnetic field,” McDonough continues.
Modeling the Solar System’s Early Years
The duo’s new model suggests that as the solar system formed, our infant star was surrounded by swirling clouds of gas and dust, within which were grains of iron. These tiny iron fragments were pulled towards the center of this maelstrom by the magnetic influence of the young star that would become our Sun.
This means that as gravity drew together gas and dust clumps not used in the formation of the Sun to form the inner planets, the protoplanets at the center closer to their parent star gathered more iron. The gravitational influence of these protoplanets resulted in these denser iron grains being drawn to their centers, thus incorporating the metal into their cores.
The duo discovered via their model that another factor determined the concentration of iron within a planet’s core aside from the proximity to its parent star that also relates to magnetic fields. The strength of the star’s magnetic field during a particular planet’s origin also determines how much iron will be deposited within its core.
This discovery could play a significant role when we are considering extra-solar planets — or exoplanets. And as the composition of a planet’s core can play a role in supporting life, these findings could influence our search for life outside the solar system.
How do planet cores help support life?
As we are only aware of life on one planet, we can only really speak about the effect the composition and state of Earth’s core have played in supporting life forms.
On our planet, a molten iron core provides conditions in which electrons are violently ripped from atoms. This generates a magnetic field that surrounds the planet — the magnetosphere.
The magnetosphere protects life here on Earth by trapping charged particles from the Sun that could quickly strip away our atmosphere, ensuring they travel down field lines and pass harmlessly out in the wake of our homeworld without hitting the surface or the atmosphere.
A striking example of what happens to a planet that isn’t afforded protection from charged particles from the Sun by a magnetosphere is Mars. The Red Planet’s inhospitable, barren landscape is the result of its atmosphere being stripped away by charged particles in the Sun’s solar winds and by cosmic rays and other space weather.
What does this mean for the composition of exoplanets?
The model put forward by McDonough and Yoshizaki has some interesting implications for the growing field of exoplanet research. Currently, astronomers can estimate the chemical compositions of the atmospheres of such worlds as they pass their host star, but this has to be done from space. With Earth-based observations, the only way of inferring the chemical composition of a planet is simply by assessing the chemical composition of its star.
This new understanding of the role of magnetic fields in the composition of planet cores means that astronomers may no longer be able to infer the chemical composition of a planet from that of its parent star.
You can no longer just say, ‘Oh, the composition of a star looks like this, so the planets around it must look like this. Now you have to say, ‘Each planet could have more or less iron based on the magnetic properties of the star in the early growth of the solar system.’
William McDonough, Professor of Geology, University of Maryland
To test their model, the duo of scientists will know set about searching for a system of planets similar to the solar system with rocky bodies existing at wide radii from a central star.
They will then assess how the density of these worlds change as the distance from their parent star increases, expecting that they will find the two factors are inversely proportional. Finding planets closer to a host star are denser than those further out could help support their new model and confirm the role magnetic fields play in core composition.
Disclaimer: The views expressed here are those of the author expressed in their private capacity and do not necessarily represent the views of AZoM.com Limited T/A AZoNetwork the owner and operator of this website. This disclaimer forms part of the Terms and conditions of use of this website.
Source:
1. McDonough. W. F., Yoshizaki. T., [2021], ‘Terrestrial planet compositions controlled by accretion disk magnetic field,’ Progress in Earth and Planetary Science, [https://doi.org/10.1186/s40645-021-00429-4]