Scientists at Rice University have found a phase-changing quantum material and a way to find more of them. This material has the potential to be used to develop flash-like memory that can store quantum bits of information, or qubits, even when a quantum computer is not in use.
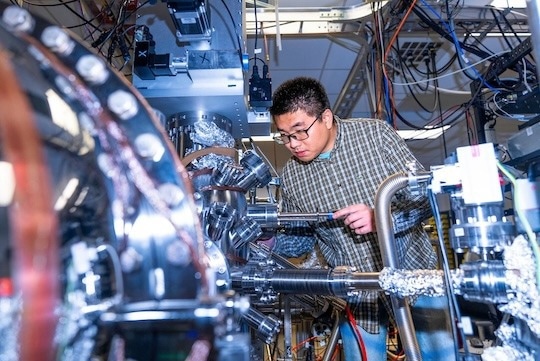
Rice University graduate student Han Wu conducting an angle-resolved photoemission spectroscopy experiment in Rice’s Quantum Materials Spectroscopy Laboratory. Image Credit: Gustavo Raskosky/Rice University.
Non-volatile digital memory that is sold commercially has phase-changing materials in it. For instance, a laser is used to heat minute particles of material in rewritable DVDs, and when the material cools, it either crystallizes or forms amorphous clumps. Bits of digital information are stored as ones and zeros in two phases of the material with very different optical properties.
In a recent open-access study that was published in Nature Communications, Ming Yi, a Rice Physicist, and over 30 co-authors from 12 different institutions demonstrated that they could switch an iron, germanium, and tellurium crystal between two electronic phases using heat.
In all of these, topologically protected quantum states are generated by the restricted mobility of electrons. Storing qubits in topologically safe states may help to lessen the errors connected to decoherence that have dogged quantum computing.
This came completely as a surprise. We were initially interested in this material because of its magnetic properties. But then we would conduct a measurement and see this one phase, and then for another measurement we would see the other. Nominally it was the same material, but the results were very different.
Ming Yi, Physicist, Rice University
It took more than two years and collaborative work with dozens of colleagues to decipher what was happening in the experiments. After heating the crystal samples before the experiments, the researchers discovered that some of them had cooled more quickly than others.
Yi and colleagues discovered that the iron-germanium-tellurium alloy switched phases without the need for melting and recrystallization, in contrast to the materials utilized in the majority of phase-changing memory technologies. Instead, they discovered that the order of vacancies - empty atomic sites - in the crystal's lattice varied according to the rate of crystal cooling. They demonstrated that they could easily reheat the crystal and cool it for either a longer or shorter duration to transition between the two patterned phases.
If you want to change the vacancy order in a material, that typically happens at much lower temperatures than you’d need to melt everything.
Ming Yi, Physicist, Rice University
Only a few studies, according to Yi, have looked into how variations in vacancy order affect the topological characteristics of quantum materials.
Yi said, “That’s the key finding,” she said of the material’s switchable vacancy order. “The idea of using vacancy order to control topology is the important thing. That just hasn’t really been explored. People have generally only been looking at materials from a fully stoichiometric perspective, meaning everything’s occupied with a fixed set of symmetries that lead to one kind of electronic topology. Changes in vacancy order change the lattice symmetry. This work shows how that can change the electronic topology. And it seems likely that vacancy order could be used to induce topological changes in other materials as well.”
I find it amazing that my experimentalist colleagues can arrange a change of crystalline symmetry on the fly. It enables a completely unexpected and yet fully welcoming switching capacity for theory as well as we seek to design and control new forms of topology through the cooperation of strong correlations and space group symmetry.
Qimiao Si, Study Co-Author and Theoretical Physicist, Rice University
The lead authors of the study are Han Wu and Lei Chen, both of Rice. Additional Rice co-authors include Jianwei Huang, Xiaokun Teng, Yucheng Guo, Mason Klemm, Chuqiao Shi, Chandan Setty, Yaofeng Xie, Bin Gao, Junichiro Kono, Pengcheng Dai, Yimo Han and Si. Yi, Dai, Han, Kono, and Si are each members of the Rice Quantum Initiative and the Rice Center for Quantum Materials.
The research was co-authored by researchers from the University of Washington, Los Alamos National Laboratory, South Korea’s Kyung Hee University, the University of Pennsylvania, Yale University, the University of California Davis, Cornell University, the University of California Berkeley, the Stanford Linear Accelerator Center National Accelerator Laboratory, Brookhaven National Laboratory and Lawrence Berkeley National Laboratory.
This research was funded by the Department of Energy (DOE) Office of Science User Facilities, the DOE Office of Basic Energy Sciences, the Gordon and Betty Moore Foundation’s EPiQS Initiative, the Robert A. Welch Foundation, the Air Force Office of Scientific Research, a Vannevar Bush Faculty Fellowship managed by the Office of Naval Research on behalf of the Department of Defense Basic Research Office.
DOE National Nuclear Security Administration, the DOE Laboratory Directed Research and Development Program, the Army Research Office, the National Science Foundation, the Alfred P. Sloan Foundation’s Sloan Research Fellows Program, and Rice’s Electron Microscopy Center also funded the study.
Journal Reference:
Wu H., et al. (2024) Reversible non-volatile electronic switching in a near-room-temperature van der Waals ferromagnet. Nature Communications. doi.org/10.1038/s41467-024-46862-z