By Ankit SinghReviewed by Susha Cheriyedath, M.Sc.Jul 11 2024
Quantum magnetism, a field at the intersection of quantum mechanics and magnetism, investigates the magnetic properties of materials where quantum effects play a significant role. This discipline has evolved from classical magnetism to incorporate quantum mechanics, leading to the discovery of novel magnetic phases and exotic quantum states.
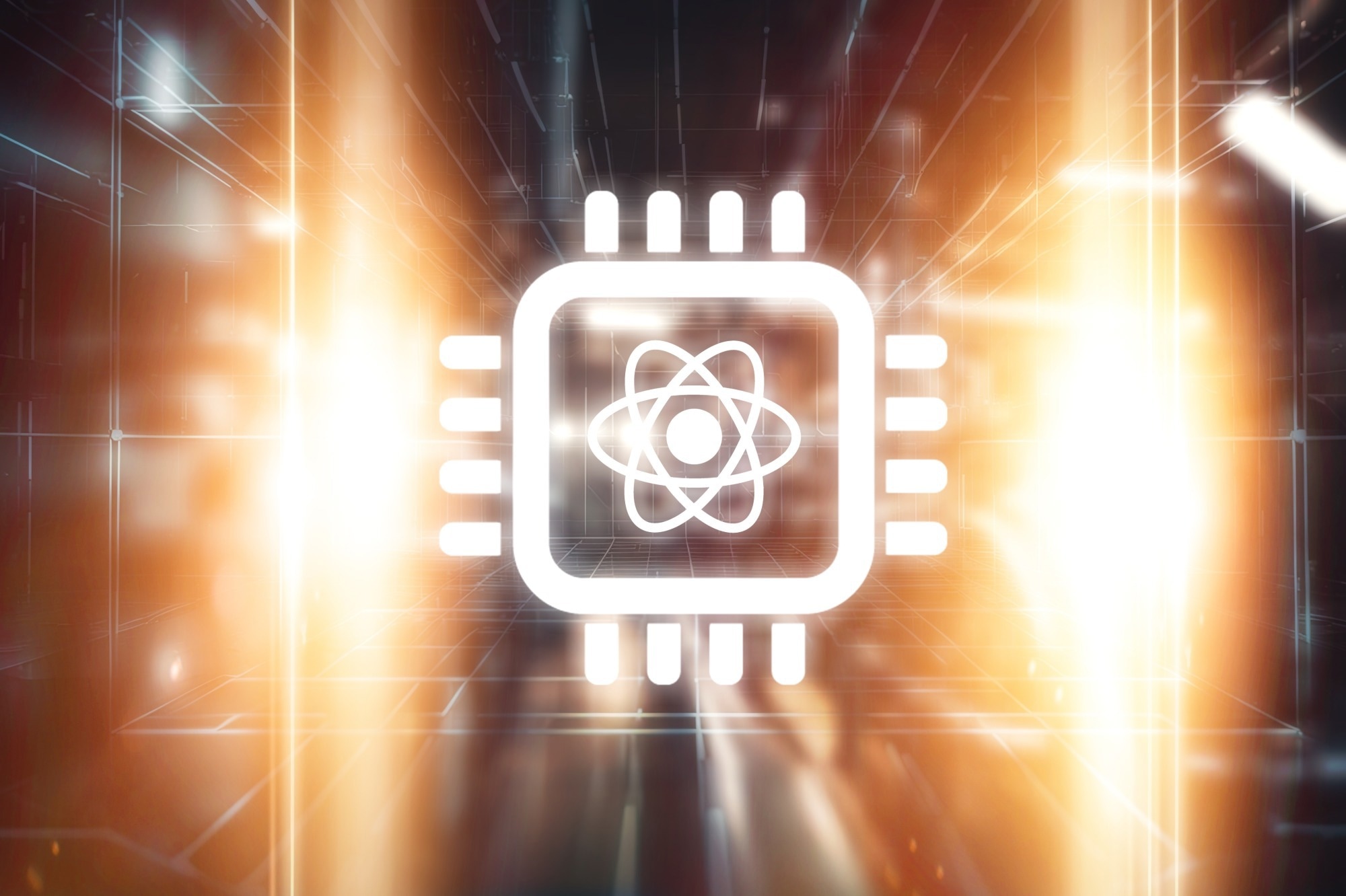
Image Credit: Billion Photos/Shutterstock
Recent advancements in quantum magnetism have greatly enhanced our understanding of material properties, opening the door to innovative technological applications. The study of quantum magnetism involves analyzing the interactions of quantum spins in various materials. These interactions can manifest in unique ways, influenced by the material’s structural properties and environmental factors. This article will explore how these quantum behaviors contribute to new possibilities in technology and science.
Historical Evolution
The study of magnetism dates back to ancient times, with early observations of naturally magnetized materials like lodestone. In the 19th century, classical theories by Ampere and Maxwell provided a macroscopic understanding of magnetic phenomena.
However, the advent of quantum mechanics in the early 20th century revolutionized our understanding at a microscopic level. Quantum theory introduced the concept of spin, a fundamental property of electrons that contributes to magnetic moments. This advancement led to the development of quantum magnetism, which focuses on the behavior and interactions of spins in various materials.
The field has witnessed significant milestones, including the discovery of quantum Hall effects and high-temperature superconductivity, both deeply connected to quantum magnetic phenomena. These discoveries have expanded our understanding and highlighted the complexity and richness of quantum magnetic systems, spurring further research into more complex materials and phenomena.1
Quantum Mechanics and General Relativity
Principles of Quantum Magnetism
Quantum magnetism is governed by the interactions between quantum spins within a material, leading to various magnetic phases such as ferromagnetism, antiferromagnetism, and spin liquids. Quantum entanglement and superposition both play a crucial role in this regard, facilitating phenomena like quantum tunneling and spin coherence. Key theoretical models, including the Heisenberg and Ising models, describe these spin interactions and help predict magnetic behaviors under different temperatures and external conditions.1
Moreover, advanced techniques like neutron scattering, magnetic resonance, and synchrotron radiation are crucial in studying quantum magnetic systems. These methods allow scientists to delve into the microscopic details of spin interactions and dynamics, offering deeper insights into the fundamental mechanisms of quantum magnetism. The development of sophisticated computational models also plays a vital role in simulating complex quantum magnetic phenomena, significantly enhancing our understanding.
Unconventional Magnetism in Sr2RuO4
In a recent study published in Nature Communications, researchers unveiled a new type of magnetism at the surface of the iconic material Sr2RuO4. This study revealed unconventional magnetic properties arising from the material's complex electronic structure, challenging traditional magnetic theories and opening avenues for exploring new magnetic states in other materials. It also suggests that the surface of Sr2RuO4 hosts unique magnetic interactions not observed in its bulk form, potentially due to altered symmetry and electronic correlations at the surface.2
This discovery has important implications for spintronics, where surface properties can be used to create advanced magnetic devices. The ability to control surface magnetism could enable new capabilities in magnetic sensors and memory, improving their performance and efficiency. Moreover, the findings inspire further investigations into other materials with similar surface phenomena, broadening the scope of research in quantum magnetism.2
Quantum Magnetism on Small-World Networks
A recent Physical Review B article involved studying quantum magnetism on small-world networks. This research revealed that incorporating long-range interactions into a one-dimensional spin chain can simulate small-world network behaviors, resulting in unique magnetic properties.
This study underscores engineered networks' potential in deepening our understanding of quantum magnetic systems and their implications for quantum computing. By simulating small-world networks, researchers can investigate how long-range correlations and entanglements influence magnetic properties, offering new insights into the complexities of quantum systems.3
This approach also offers a novel platform for investigating phase transitions and critical phenomena in quantum magnetic systems. Small-world networks can exhibit enhanced connectivity and robustness, which are beneficial for developing fault-tolerant quantum devices. The findings can help design more efficient quantum communication networks and improve quantum computer scalability.3
Intersublattice Entanglement Entropy in Antiferromagnets
Another breakthrough study published in Physical Review B has focused on intersublattice entanglement entropy in antiferromagnets. The research demonstrates that entanglement entropy, which measures the quantum correlations between different parts of a system, exhibits extensive behavior in antiferromagnets. These findings provide deeper insights into the quantum nature of magnetic phase transitions and the role of entanglement in magnetic materials. Understanding entanglement entropy is vital for exploring quantum critical points and topological phases and is crucial for the development of new quantum technologies.4
Additionally, the study underscored the significance of quantum information theory in the comprehensive description of magnetic systems. By leveraging the concepts from quantum information, researchers can gain deeper insights into the intricate entanglement structure and dynamics within antiferromagnetic materials. This knowledge can be leveraged to design innovative materials with tailored magnetic properties, potentially leading to groundbreaking advancements in quantum sensing and information processing applications.4
THz Spectroscopy of Quantum Materials
Recent progress in THz spectroscopy has significantly impacted the study of quantum magnetism. THz radiation, with its ability to probe low-energy excitations, has been used to investigate magnetic and topological materials. This technique has revealed new magnetic states and dynamics, enhancing our understanding of quantum magnetic phenomena at the microscopic level. THz spectroscopy enables the direct observation of spin and charge dynamics, providing valuable information about the interactions and couplings in quantum materials.5
The use of THz radiation has also facilitated the study of ultrafast magnetic processes, allowing researchers to explore the transient behaviors and nonequilibrium states in quantum magnetic systems. These insights are crucial for developing ultrafast spintronic devices and understanding the fundamental limits of magnetic switching speeds. The advancements in THz spectroscopy thus contribute to both fundamental research and practical applications in quantum magnetism.5
Non-Cryogenic Quantum Magnetic Sensors
Quantum magnetic sensors that operate at room temperature represent a significant advancement. These sensors leverage quantum phenomena, such as spin coherence and entanglement, to achieve high sensitivity and precision. The ability to function without cryogenic cooling reduces the cost and complexity of these sensors, enabling more widespread use in diverse applications, including medical imaging and environmental monitoring.6
Non-cryogenic sensors are particularly valuable for detecting weak magnetic fields, which is essential for biomagnetism and geophysics applications. The high sensitivity of these sensors enables the detection of minute magnetic signals, providing detailed information about biological processes and subsurface structures. As a result, non-cryogenic quantum magnetic sensors have the potential to revolutionize various fields, from healthcare to natural resource exploration.6
Spintronics and Quantum Computing
Quantum magnetism has also significantly contributed to spintronics and quantum computing. Recent studies have focused on manipulating spin states to store and process information, allowing for the development of spin-based quantum bits (qubits). These advancements promise faster and more efficient computing technologies, with potential applications in cryptography and complex simulations. Spintronics uses the spin degree of freedom of electrons, offering various advantages such as non-volatility, high-speed operation, and low power consumption.7
The integration of spintronics with quantum computing has led to the concept of quantum spintronics, where spin states are used to perform quantum computations. This hybrid approach combines the best features of both fields, aiming to create robust and scalable quantum computing architectures.7
Magnetic Skyrmions
Magnetic skyrmions, nanoscale spin structures, have gained considerable attention due to their stability and potential for data storage. Recent research has explored the creation and manipulation of skyrmions using electric fields and thermal gradients. These advancements could lead to high-density, low-power memory devices and novel spintronic applications. Skyrmions exhibit topological protection, meaning they resist perturbations and defects, making them ideal for reliable data storage.8
The capacity to regulate skyrmion dynamics through external stimuli like electric fields provides a flexible and energy-efficient approach to data processing. Researchers are also investigating the use of skyrmions in neuromorphic computing, where their unique properties can be used to mimic the behavior of neurons and synapses. This advancement may result in the creation of artificial neural networks with improved efficiency and performance.8
Challenges in Quantum Magnetism
Despite the numerous advancements, several challenges persist in the field of quantum magnetism. One of the primary challenges is the precise control and manipulation of quantum spins in materials. Achieving and maintaining coherent spin states at practical temperatures and conditions remain difficult. Moreover, understanding and mitigating decoherence effects, which cause loss of quantum information, is critical for the development of reliable quantum devices.
Another significant challenge is the integration of quantum magnetic materials into existing technologies. The fabrication and scalability of quantum devices using these materials need substantial improvement. Furthermore, the theoretical models and computational methods required to accurately describe complex quantum magnetic systems are still in development. Addressing these challenges requires continued interdisciplinary research and collaboration, combining expertise in physics, materials science, and engineering.
Future Prospects and Conclusions
The future of quantum magnetism is promising, marked by steady advancements in experimental and theoretical research. This progress is paving the way for significant developments in technologies such as quantum sensors and spintronic devices, which benefit greatly from a deeper understanding of quantum magnetic phenomena. Moreover, the integration of quantum magnetism with other areas of quantum technology is poised to make substantial contributions to fields ranging from computing to materials science.
In conclusion, the recent advancements in quantum magnetism underscore the dynamic nature of this field. By applying quantum mechanics to investigate and control magnetic properties, researchers are discovering new physical phenomena and enabling innovative technological applications. Ongoing interdisciplinary research and collaboration will be crucial to realizing the full potential of quantum magnetism in the future.
References and Further Reading
- Higemoto, W. et al. (2022). Direct measurement of the evolution of magnetism and superconductivity toward the quantum critical point. Proc. National Acad. Sci, 119 (49). DOI: 10.1073/pnas.2209549119
- Fittipaldi, R. et al. (2021). Unveiling unconventional magnetism at the surface of Sr2RuO4. Nat. Commun, 12 (1). DOI: 10.1038/s41467-021-26020-5
- Dupont, M.; Laflorencie. (2021). N. Quantum magnetism on small-world networks. Phys. Rev. B, 103 (17). DOI: 10.1103/physrevb.103.174415
- Hartmann, D. M. F.et al. (2021). Intersublattice entanglement entropy as an extensive property in antiferromagnets. Phys. Rev. B, 104 (6). DOI: 10.1103/physrevb.104.064436
- Bera, A. et al. (2021). Mondal, M. Review of recent progress on THz spectroscopy of quantum materials: superconductors, magnetic and topological materials. Eur. Phys. J. Spec. Top. DOI: 10.1140/epjs/s11734-021-00216-8
- Ohkubo, M. (2023). The emergence of non-cryogenic quantum magnetic sensors: Synergistic advancement in magnetography together with SQUID. Rev. Sci. Instrum, 94 (11). DOI: 10.1063/5.0167372
- Dey, P., Roy, J.N. (2021). An Overview of Spintronics. In: Spintronics. Springer, Singapore. DOI: 10.1007/978-981-16-0069-2_1
- Li, S. et al. (2021). Magnetic skyrmions for unconventional computing. Mater. Horiz. DOI: 10.1039/d0mh01603a
Disclaimer: The views expressed here are those of the author expressed in their private capacity and do not necessarily represent the views of AZoM.com Limited T/A AZoNetwork the owner and operator of this website. This disclaimer forms part of the Terms and conditions of use of this website.