New and inventive methods to create, control and manipulate qubits are being pursued across the rapidly expanding quantum technology industry. Recent experiments using two-dimensional (2D) materials - specifically Van der Waals materials - as a qubit platform, have demonstrated coherent, sustainable qubit operations using nuclear spins at room temperature.
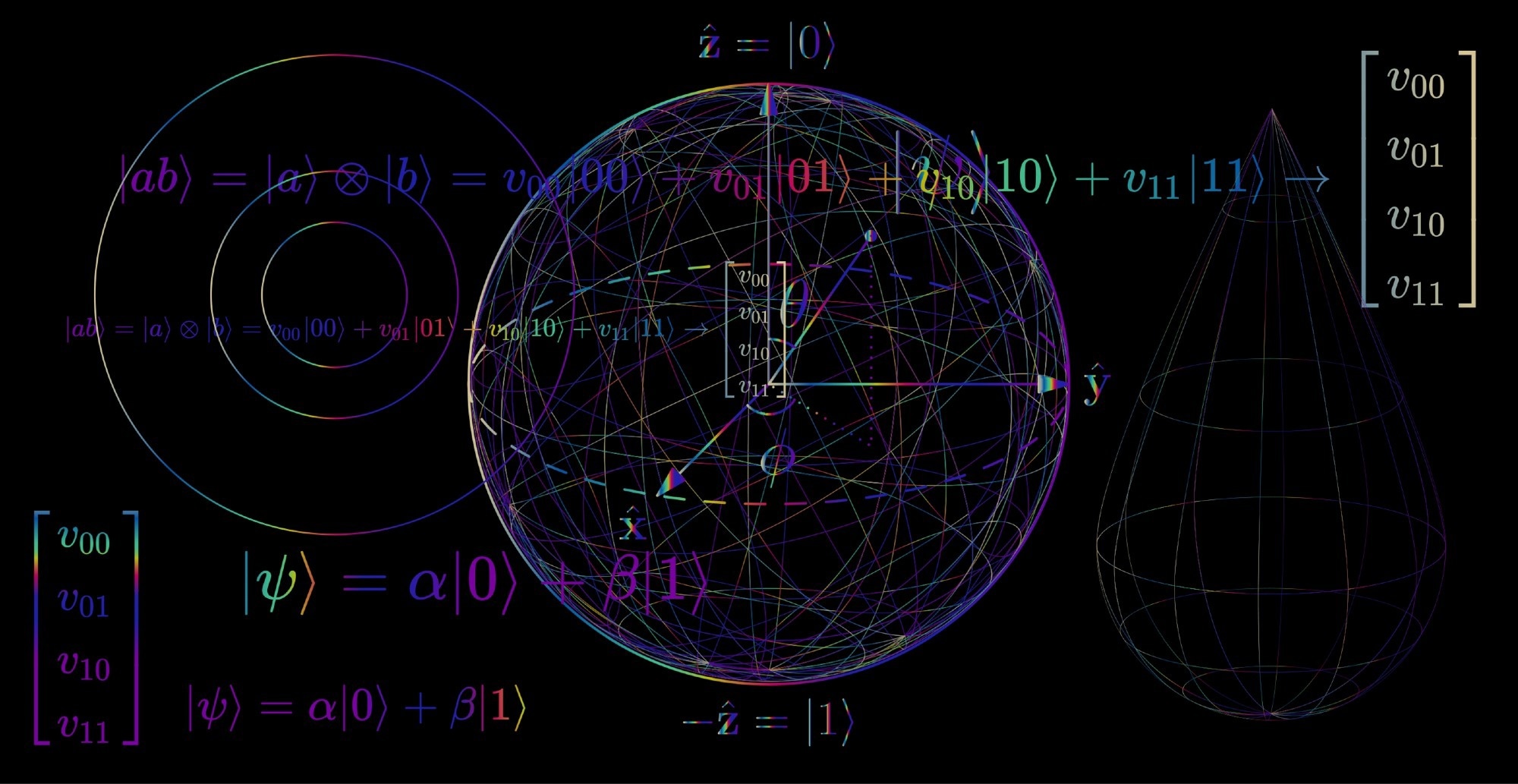
Image Credit: local_doctor/Shutterstock.com
Quantum technology is on the verge of transforming how the world operates. The next phase of computing, sensing, and communications are expected to rely on the controlled quantum behavior of matter. In the quest to control the “strange” properties of quantum mechanics, like superposition and entanglement, different technological platforms have been proposed. Some examples are neutral atom arrays, trapped atomic or molecular ions, and superconducting qubits. These approaches use properties of photons emitted from particular atomic transitions or electronic spins to initiate qubits and encode and manipulate information.
One of the main drawbacks of the qubit generation approaches mentioned above is that they have to be operated in ultracold temperatures. Condensed matter-based platforms have emerged as a promising alternative that can perform well at room temperatures.
Van der Waals Materials
2D materials offer a unique, solid-state system to study quantum effects. 2D materials are made of crystalline sheets of atoms layered vertically. They exhibit stronger interatomic interaction on the planer surface than between vertical layers. Within the umbrella of 2D materials, individual atomic planes bound together by weak van der Waals (vdW) forces are called vdW materials. These materials have optical and electronic properties that are similar to insulators, metals and semiconductors combined. By exploiting these qualities using lasers and Nano-photonic techniques, many quantum phenomena can be studied.
Hexagonal Boron Nitride
Hexagonal Boron Nitride (hBN) has grown in prominence among vdW materials due to its optical and fabrication versatility. hBN is composed of boron and nitrogen atoms. The physical properties of hBN depend on how the atoms are arranged within the bulk molecular structure.
Defects fabricated within hBN structures make them optically active. They also provide the opportunity to be controlled with external eclectic and magnetic fields to manipulate their polarizations and other properties. Negatively charged boron vacancy can be fabricated on a bulk structure using different methods. For example, monocrystalline hBN can be irradiated by a neutron beam at specific thermal fluxes to produce a negatively charged boron vacancy surrounded by symmetric nitrogen atoms as shown in figure 1. This specific patterning of hBN has allowed experimentation with nuclear spin control.
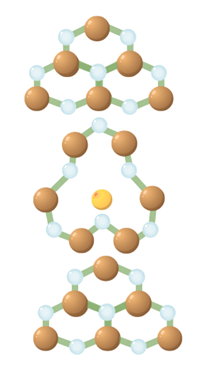
Figure 1: Schematic of boron vacancy in hBN: a negatively charged boron vacancy (yellow sphere) is in the middle of nitrogen atoms (silver spheres). Brown spheres denote boron atoms.
While many recent investigations involving vdW materials have relied on using electronic spins, nuclear spins have been largely untapped as a quantum resource.
Nuclear Spin
Qubits for quantum technology are largely created by exploiting the internal structure and properties of atoms. When considering the internal make-up of an atom, electrons have intrinsic angular momentum called spins. These are commonly used to encode quantum information. The nucleus of the atom also possesses a nuclear spin due to its intrinsic angular momentum. Controlling the nuclear spin has been far more challenging than using electronic properties.
In hBN defects, nuclear spins can be initiated and detected by manipulating the transitions created by the vacancy. The electron spin qubits which surround the nitrogen nucleus are optically addressed to set the nuclear spins to a known initial state. Polarization of the electronic system selectively aligns the spin properties of the nucleus.
Optically Initiating the Nuclear Spins
When the hBN vacancy is created, the nuclear spins of the three nitrogen atoms around the negative vacancy are in a random state of -1, 0 or +1. Optical pumping is employed by a laser to excite the negatively charged defect to a higher energy state. The laser radiation does not directly affect the nuclear spins. The state of the nuclear spin in the nitrogen nucleus is forced to flip due to the excited state electron. Several iterations of optical pumping and readout guarantee that the nuclear spins of the nitrogen atoms are initialized correctly. Once initiated, the spins can be interrogated by rf probes for writing and reading information. They can be used as qubits and applied to develop various tasks.
Improvements in Performance
Experiments conducted using nuclear spins of hBN defects have shown enhanced control properties for qubits. VdW materials lend themselves to be manipulated under ambient temperatures. Experimental results obtained using indirectly initialized nuclear spins showed coherent performance that was 350 times better than previous measurements.
Additionally, electron-mediated nuclear-nuclear spin coupling was observed to be five times enhanced as compared to direct nuclear-spin dipolar coupling. Coherence times that are 30 times longer than electronic spin-controlled qubits were also shown with this system. This result shows that multi-qubit operations can be achieved using nuclear spins in vdW structures.
More from AZoQuantum: The James Webb Space Telescope Zooms in on Jupiter
References and Further Reading
Gao, X., Vaidya, S., Li, K. et al. Nuclear spin polarization and control in hexagonal boron nitride. Nat. Mater. (2022). https://doi.org/10.1038/s41563-022-01329-8
Xiaodan Lyu, Qinghai Tan, Lishu Wu, Chusheng Zhang, Zhaowei Zhang, Zhao Mu, Jesús Zúñiga-Pérez, Hongbing Cai, Weibo Gao. Strain Quantum Sensing with Spin Defects in Hexagonal Boron Nitride. Nano Letters 2022, DOI: 10.1021/acs.nanolett.2c01722
Gottscholl, A.; Diez, M.; Soltamov, V.; Kasper, C.; Sperlich, A.; Kianinia, M.; Bradac, C.; Aharonovich, I.; Dyakonov, V. Room temperature coherent control of spin defects in hexagonal boron nitride. Sci. Adv. 202, 7, DOI: 10.1126/sciadv.abf3630
Disclaimer: The views expressed here are those of the author expressed in their private capacity and do not necessarily represent the views of AZoM.com Limited T/A AZoNetwork the owner and operator of this website. This disclaimer forms part of the Terms and conditions of use of this website.