AZoQuantum interviewed Dr. Samuli Autti from Lancaster University about his research that has explored quantum time crystals. In his latest paper, Dr. Autti sheds light on previously misunderstood phenomena.
Could you please introduce yourself and how you came to be involved in this research?
My name is Samuli Autti, and I am an EPSRC Fellow at Lancaster University in the United Kingdom. My Ph.D., which I finished five years ago in Finland, contained some of the very first time crystal experiments, although we did not understand that back then.
Can you explain what a time crystal is?
A time crystal is a material where the constituent particles keep moving synchronously in a repeating pattern in the absence of external encouragement. If this sounds like a perpetual motion machine, you can congratulate yourself: it is a perpetual motion machine, and therefore time crystals are impossible. No wonder physicists are so interested in creating them...
How do time crystals differ from standard crystals?
In a crystalline solid (“space” crystal), the same arrangement of atoms is repeated over and over in all directions. In a time crystal, the repetition happens in time. For example, at odd seconds all atoms in the time crystal are moving left, and at even seconds they are moving right. So the name “crystal” is a mathematical reference to repetition. That is, a time crystal does not need to be solid at all, and our time crystals are actually liquid.
What are some of the problems associated with creating this state of matter?
Everybody knows that perpetual motion machines do not exist. A time crystal is a perpetual motion machine. Are time crystals therefore impossible? Not so fast! It turns out that in quantum physics perpetual motion is no problem, as long as it cannot be observed. So, we can create a time crystal and it may keep moving as long as we are not looking at it, but it must start to slow down when we open our eyes.
Why would time crystals be useful in quantum computers?
Many of the difficulties people face with building quantum computers are connected to the issue of looking and not looking at a time crystal, especially with how to switch from one of these to the other one smoothly. On the other hand, quantum computers in development have recently been used as platforms to study the formation of a time crystal in slow motion.
Now, it may be difficult to predict how the two concepts will eventually intertwine: one field may turn into technology for supporting the other one; or we could learn that quantum computers are impossible because they hit classical physics when made bigger, and that this barrier is related to how time crystals melt, for example.
Could you explain your procedure for creating time crystals?
This will be a bit technical. The time crystal is created in superfluid helium-3. Helium-3 is a rare version of helium with one neutron less than in the usual helium-4 that we put in balloons. It becomes superfluid at about one-thousandth of a degree above absolute zero.
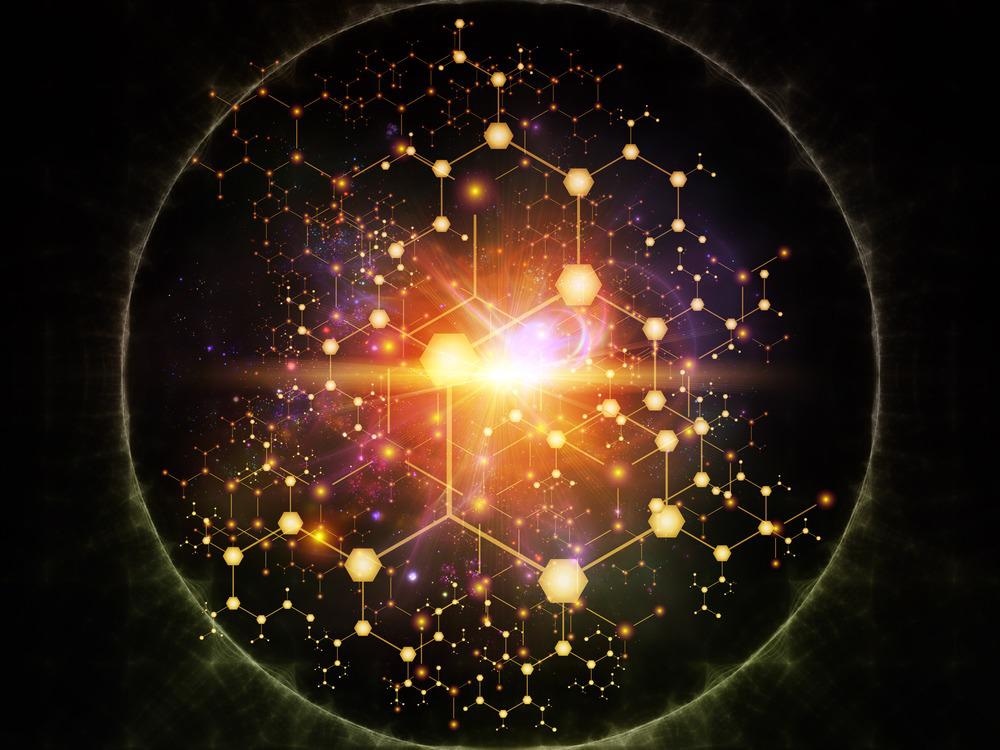
Image Credit: agsandrew/Shutterstock.com
The experiment we carried out requires an even lower temperature, about 1/10000 of a degree above absolute zero. To reach these low temperatures is difficult but can be done in a specialized research laboratory. Then we simply throw suitable radio waves from an aerial in the direction of the superfluid to create the time crystals and stay around listening for a returning transmission to know what the time crystals did. In the end, the time crystal perpetual motion looks like an oscillating wave on a computer screen.
Can you explain how these crystals interacted? What phenomena associated with quantum physics did they demonstrate?
If time crystals can only exist when we are not looking, how about two time crystals interacting, should that not be impossible as well (one time crystal “looking” at the other one)? This is what our most recent experiment was about, and we have shown that such interaction can be arranged and it causes no trouble!
We made two time crystals touch. In quantum physics this leads to particles moving between the time crystals in such a way that the time crystal that is "behind" in the pattern of repeating motion receives more particles. We also allowed the time crystal frequencies to change. If the frequencies are brought close to one another, the frequencies start repelling each other, and a large number of constituent particles move between the time crystals abruptly. These are typical features of a two-body system in quantum physics and observing them confirms that the time crystals are interacting as predicted by quantum physics.
Are there any other applications besides quantum computers that these time crystals could be used in?
Quantum computers are a fashionable field, but they are only one example of exploiting the different rules of the quantum world. Research laboratories use many instruments and devices that are based on or only possible because of quantum physics, and new ones are being invented all the time.
One of the basic building blocks in a quantum device is a “two-level system”, and we have shown that two time crystals make such a system. To turn quantum devices into everyday technology, they first need to work in ambient conditions instead of extremely low temperatures. Curiously, time crystals similar to the ones we study have recently been created in an “on-chip system” that works at room temperature. This system may be more challenging to control than an ultra-cold time crystal due to unwanted interference from the hot environment, but I am confident our colleagues will eventually get there. So maybe one day time crystals will be a component in your smartphone or some other everyday device.
Was there anything about the study you conducted that surprised you or your colleagues?
Certainly there were many things, but I am still astonished at how long it took us to understand what the experimental results meant. The first measurements were done a decade ago, and only now do we properly understand them.
What is next for this research?
We are going to make the time crystals touch a large mechanically moving object to learn how such interaction affects the time crystals. If we are lucky, we will also learn something fundamental about the superfluid itself by doing this. In the longer perspective, the plan is to probe the edges of the new time crystal phase to find out, for example, what happens if we melt a time crystal.
Where can readers find more information?
The research article is free to read and can be found here https://www.nature.com/articles/s41467-022-30783-w
About Dr. Samuli Autti
Samuli Autti is a Lecturer and EPSRC Fellow at Lancaster University in the UK, and a leading superfluid scientist of his generation. Before moving to Lancaster, he completed a Ph.D. at Aalto University in Finland in 2017 on ultra-low temperature physics. Samuli has authored many field-defining discoveries on large-scale quantum systems. Examples are light Higgs boson quasiparticles and their interactions (2015), half-quantum vortices (2016), and time quasicrystals and time crystal interactions (2018-present). His present work deals with the interfaces between classical and quantum physics, aiming to answer questions such as “what does it feel like to touch a quantum fluid” and “can we melt a time crystal, and whether the outcome is a time liquid”.
Interview questions were provided in part by Robert Lea.
Disclaimer: The views expressed here are those of the interviewee and do not necessarily represent the views of AZoM.com Limited (T/A) AZoNetwork, the owner and operator of this website. This disclaimer forms part of the Terms and Conditions of use of this website.