This article explores the need for photon counting technologies in future projects as well as potential technological options for meeting those objectives. The utility of semiconductor and superconducting technologies towards the counting of photons in the vacuum ultraviolet to short-wavelength infrared is also discussed.
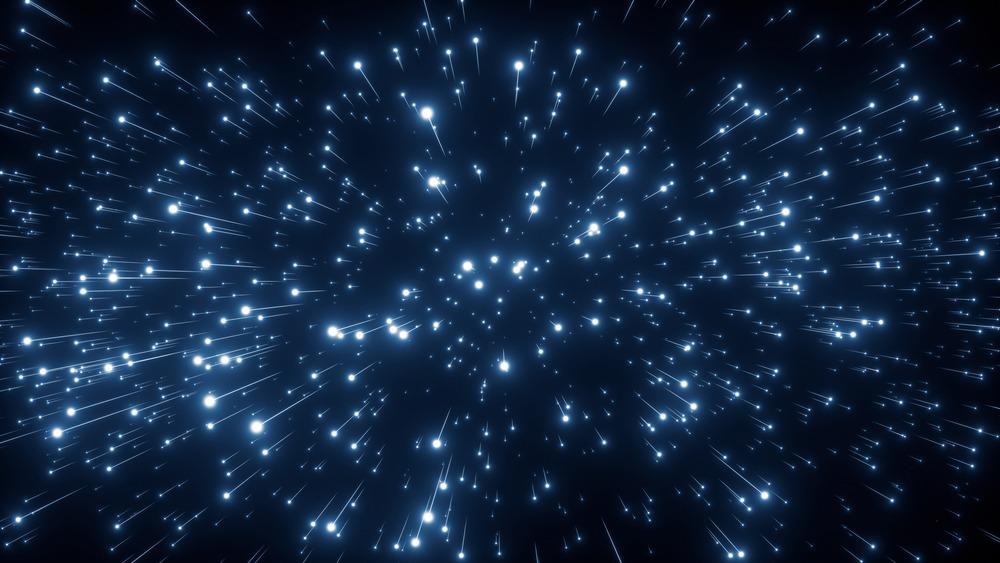
Image Credit: S.Gvozd/Shutterstock.com
Several photon counting devices have been developed in the previous decade, allowing for new studies in the low photon number zone. These discoveries will assist a number of current and future high energy physics (HEP) projects, as well as have a significant influence outside of HEP. There is a substantial technological possibility to fully develop these sensors and have a significant effect on HEP during the next decade.
Applications of Photon Counting Detectors
Detector readout noise currently limits ground-based spectroscopic investigations of dim astronomical objects in the low-signal, low-background range. As a result, the ability to dynamically regulate readout noise will allow photon counting for dim sources when needed. Observing campaigns (spectroscopy or imaging) that consist of multiple short-duration exposures is another application for single-photon counting.
For today's ground-based observatories, scientific charged coupled devices (CCDs) are the detector of choice. Current scientific CCDs have a noise floor of 2 e- rms/pix.
The new generation of silicon CCDs with ultralow readout noise is among the most suitable detector technologies for the building of a massive multi-kg experiment for the probing of electron recoils from sub-GeV dark matter (DM). The Sub-Electron Noise Skipper-CCD Experimental Instrument SENSEI collaboration proved the ability to precisely measure the quantity of free electrons in every pixel across the CCD. SENSEI built a small skipper-CCD prototype sensor and collected data on the surface and underground at Fermilab, establishing world-leading bounds on DM-electron interactions for the DM masses ranging from 500 keV to 5 MeV.
Due to their inherent low energy threshold, which has been experimentally demonstrated to be 125 meV, superconducting nanowire single-photon detectors (SNSPDs) also hold potential for the detection of light dark matter. This could enable sensitivity to masses less than 0.1 MeV when dark matter generates an electron recoil which leads to scintillation in a cryogenic semiconductor. Large-scale noble element-time projection chambers (TPCs) play a key part in both of these fields of neutrino research, and by improving their capabilities, they can now produce discovery-level measurements.
Although silicon can absorb photons up to 1110 nm, utilizing it in structures larger than 800 nm necessitates thick structures with a long and homogenous electric field. In order to achieve practical sensitivity in the short-wave infrared (1 to 3 µm), semiconductors like germanium or tiny bandgap semiconductor elements must be used.
Recent research utilizes traditional CCD cameras for detection, which have high efficiency of greater than 90% and readout noise of 2 to 5 e-. At excess voltages between 2 V and 7 V and temperatures achievable with thermoelectric cooling, small diameter InGaAs/InP single-photon avalanche diodes (SPADs) were reported with dark count rates ranging from 102 cps to 104 cps.
Future Prospects
Multiplexing can be achieved by tuning each pixel to a separate resonant frequency with lithography during device construction since the resonators' quality factor Q is large and their microwave transmission off-resonance is virtually perfect. Recent improvements in the understanding of MKID's noise, along with a quantum-limited parametric amplifier, have greatly enhanced MKID spectrum resolution.
Single-photon sensitivity at wavelengths out to several microns, timing jitter as low as a few ps, dark count rates (DCR) down to 6 x 10-6 Hz, and detection efficiency (DE) of 0.98 have been reported for superconducting nanowire single-photon detectors (SNSPDs). They have also been shown to work in magnetic fields as high as 6 Tesla. Recent experiments have demonstrated that aSe can respond to vacuum ultraviolet (VUV) light in a cryogenic environment with a low applied electric field.
Summary
In this article, the need for the development of photon counting technologies in the next generation of HEP experiments has been discussed based on the latest literature. With photon-counting sensors and better infrared (IR) sensitivity, the subsequent generation corresponding to spectroscopic cosmic surveys will be more efficient.
Future space telescope missions could benefit from these technologies as well. In the dark matter and low-energy neutrino investigations, photon-counting systems have also shown world-leading performance. Furthermore, to fully exploit the potential of noble elements TPCs, high-UV-efficiency, and large-area sensors that can detect both light and charge are required.
Quantum sensing, low-energy radiation detection applications, and basic energy science research will all be impacted by the HEP advancements. Silicon photomultipliers (SiPMs) have a single-photon resolution in the visible range and can be used in photon detecting systems with very large areas, such as noble liquid detectors. At both the near IR and VUV wavelengths, research is being done to improve their sensitivity.
Photon-to-digital converters (PDCs) convert photon counts to digital signals in one direction and have low power consumption, inbuilt signal processing, and low time jitter. In superconducting detectors, photon counting for lower energy photons and with higher time resolution is achievable. In the future decade, the further development of these potential technologies for photon counting as part of HEP is likely to have a significant impact on the field.
Reference
Asaadi, J., Baxter, D., Berggren, K. K., et al. Photon counting from the vacuum ultraviolet to the short wavelength infrared using semiconductor and superconducting technologies. Proceedings of the US Community Study on the Future of Particle Physics (Snowmass 2021). https://arxiv.org/pdf/2203.12542.pdf
Disclaimer: The views expressed here are those of the author expressed in their private capacity and do not necessarily represent the views of AZoM.com Limited T/A AZoNetwork the owner and operator of this website. This disclaimer forms part of the Terms and conditions of use of this website.