AZoQuantum talks to Dr. James Quach about his research surrounding quantum batteries and the theoretical concept of superadsorption.
Please can you introduce yourself, your background, and how you became involved with this area of research?
I am a physicist from The University of Adelaide. I become involved in this area of research because I saw the potential impact quantum batteries could have.
How do the laws of quantum physics differ from those of classical physics?
This is not a question with a short answer, but we can focus here on what is relevant for quantum batteries. One key difference is that according to quantum physics, the probability of something happening is governed by a “wave function”. Just like other waves (sound waves, waves on the ocean, etc), different waves can either add together (“constructive interference”) or cancel each other out (“destructive interference”). Crucially, this means that if there is more than one way to get from an initial state to a final state, quantum physics says that the wave function requires one to add up these different ways.
One example of this is the famous “double slit” experiment, where particles passing through two slits either add constructively or destructively, leading to variations in how likely a particle is to be found at different points behind the screen. Another example occurs in our experiment, where routes to absorbing light interfere constructively.
What are quantum batteries, and how do they operate?
Quantum batteries are energy storage devices that use the laws of quantum mechanics to improve their ability to store energy, particularly how fast they can be charged. Superabsorption is a quantum effect where different routes of molecular excitation by the absorption of a photon interfere constructively, as discussed above. Crucially, this allows combined molecules to absorb light and charge the battery more efficiently than if each molecule were acting individually.
What has limited the development of quantum batteries?
In the past, quantum batteries have only been a theoretical idea. Our work provides an important experimental proof-of-concept.
Could you please describe your research process and the development of your quantum battery model?
In our research, we experimentally proved the concept of superabsorption, a key underpinning of quantum batteries. To achieve this, we developed our test device with a ”microcavity”. A microcavity consists of two mirrors, where the spacing between the mirrors is of the order of a micrometer. The device contains an “active” layer of organic dye (i.e. carbon-based molecules) in between the mirrors.
The mirrors in this microcavity were made using a standard method to make high-quality mirrors. This is to use alternating layers of dielectric material (silicon dioxide and niobium pentoxide) to create what is known as a “distributed Bragg reflector”. This produces mirrors that reflect much more of the light than a typical metal/glass mirror. This is important as we want light to stay inside the cavity as long as possible.
The organic dye in our devices was a material with the trade name “Lumogen-F Orange”. To control the concentration of the dye (i.e. how much dye there is), the dye was dispersed within an organic polymer: polystyrene. The polystyrene does not do anything active here, it just spaces out the dye molecules.
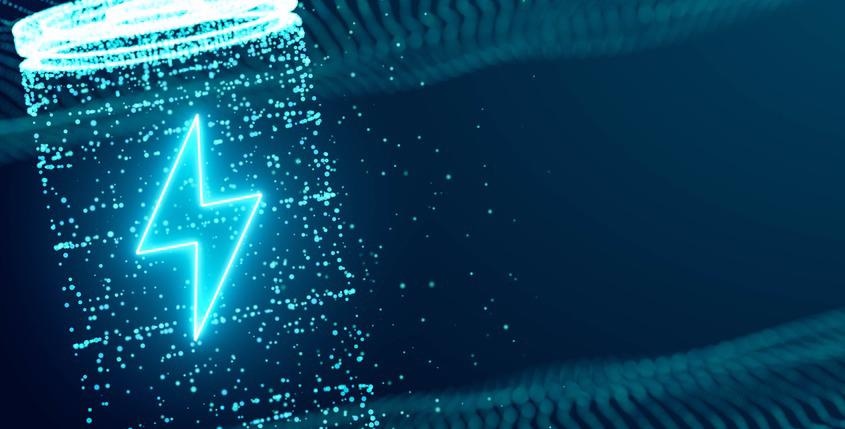
Image Credit: Black_Kira/Shutterstock.com
We then measured the charging power and capacity of our device using a special technique known as ultrafast transient absorption spectroscopy.
Battery capacity refers to how much energy can be stored, and charging power refers to how quickly the energy can be stored. Our research was mostly focused on ways to increase how fast each molecule can absorb energy, although we did also see interesting effects on the total amount of energy stored.
What is quantum decoherence and how is it relevant to your research?
Quantum coherence is the ability to maintain quantum properties. Quantum decoherence refers to the ways a quantum system loses quantum coherence as it interacts with its environment. For example, in the “two-slit” experiment described above, if information about which path a particle takes through the slits gets recorded, the interference goes away. Decoherence occurs through information about the state of the system leaking out.
Normally, decoherence is an undesirable property for quantum systems; this is why most quantum experiments require carefully isolated systems and is also related to why quantum effects are normally seen only for small systems with a few particles and go away with bigger systems.
However, in our research, we found that some decoherence actually helped stabilize the energy in quantum batteries. In particular, it serves to limit the duration over which the quantum enhancement survives. The right amount of decoherence allows a device featuring coherence-enhanced absorption of light that does not then go on to also discharge with coherence-enhanced emission.
To what extent were you able to model an improvement in recharge time and energy storage?
We were able to show both experimentally and in theoretical modeling that the charging time actually decreases as we increased the number of molecules in our device. We also showed that under some conditions the energy stored per molecule increases as we increased the number of molecules. These properties are the key underpinnings of quantum batteries.
Why are these types of batteries attracting research attention? What sorts of applications and industries are they significant to?
Quantum batteries are attracting research attention because they have the potential to significantly decrease charging times. In the same way that there has been a lot of recent investment in quantum computing—i.e. using quantum effects to make computing faster—other operations about transferring energy or even harvesting energy can in principle be made faster by using quantum effects.
The key challenge though is to bridge the gap between the proof-of-principle here for a small device and exploiting the same ideas in larger usable devices.
Was there any aspect of this research that was especially surprising or interesting to you?
The most interesting aspect of our research was that we were able to prove an important theoretical concept in the laboratory: superabsorption. A surprising aspect of our research was that we found quantum decoherence to be beneficial, in that it helped stabilize the stored energy in our device.
What are the next steps for this subject matter? Do you hope to conduct further research into quantum batteries?
The idea here is a proof-of-principle that enhanced absorption of light is possible in such a device. The next steps are to explore how this can be combined with other ways of storing and transferring energy, to provide a device that could be practically useful. We hoped to conduct further research to achieve this.
Where can readers find more information?
Readers can find our paper here: https://www.science.org/doi/10.1126/sciadv.abk3160
About Dr. Quach
Dr. Quach is a physicist at the University of Adelaide. Prior to this, he was a Marie Curie Fellow at the Institute of Photonics Science in Barcelona, and a JSPS Fellow at the University of Tokyo. He completed his Ph.D. at the University of Melbourne in Physics. Dr. Quach's research interest is in all things quantum. He works in quantum technology, quantum computing, quantum biology, quantum cognition, quantum chaos, quantum thermodynamics, and quantum gravity.
Disclaimer: The views expressed here are those of the interviewee and do not necessarily represent the views of AZoM.com Limited (T/A) AZoNetwork, the owner and operator of this website. This disclaimer forms part of the Terms and Conditions of use of this website.