AZoQuantum speaks with Dr. Richard K. Barry, Research Astrophysicist at the Laboratory for Exoplanets and Stellar Astrophysics, NASA Astrophysics Science Division. Dr. Barry has been investigating the use of AI to find masses of free-floating planets.
Can you give our readers an insight into the Contemporaneous LEnsing Parallax and Autonomous TRansient Assay (CLEoPATRA)? How was it developed and what are its aims?
In 2010, NASA received the National Research Council's Astro2010 report, New Worlds, New Horizons in Astronomy and Astrophysics. This report defined a set of science objectives for space-based astrophysical research for the coming decade. In New Worlds, New Horizons, the empaneled experts recommended that the astrophysics community build an experiment to help us understand the nature of dark energy and finish the census of all possible types of planets in our host galaxy.
Both of these goals require a spaceborne wide-field infrared observatory. NASA responded by developing the Wide-Field Infrared Survey Telescope using assets obtained from the National Reconnaissance Observatory - a retired spy satellite. This space mission, now named the Nancy Grace Roman Space Telescope (RST) - in effect a shotgun wedding of two disparate astronomical goals - is scheduled for launch in 2025.
RST will use gravitational microlensing to detect new planets around other stars. The principal observable for microlensing is, in the case of planets gravitationally bound to their host star, the ratio of the mass of the planet to the mass of the star - the mass of the planet and star and their separation being degenerate with distance from the observer. Other information is needed to break this degeneracy - either through detection of the color of the host star, a proxy for its mass, or to determine the distance to the lensing system using gravitational parallax.
Microlensing a Rogue Planet
This animation illustrates the concept of gravitational microlensing with a rogue planet — a planet that does not orbit a star. When the rogue planet appears to pass nearly in front of a background source star, the light rays of the source star become bent due to the warped space-time around the foreground planet. This planet is then a virtual magnifying glass, amplifying the brightness of the background source star. Video Credit: NASA Video / YouTube
We conceived of Contemporaneous LEnsing Parallax and Autonomous TRansient Assay, CLEoPATRA, as a small, purpose-built space mission to conduct simultaneous microlensing observations of the RST field of regard (FOR) at a great distance from Earth beginning in 2025.
CLEoPATRA - or CLEo, for short - with a 20-30 cm primary optic, will launch as a rideshare payload with another experiment already manifested for a gravitationally bound orbit about Mars. CLEo will be deployed by the launch vehicle while en route and will continue past Mars on a heliocentric orbit, providing a long physical baseline for these measurements.
CLEoPATRA’s observations have the potential to increase the precision and total number of exoplanetary mass estimates obtained by RST - one of this NASA flagship’s two primary scientific goals.
__
There are several different ways to detect planets. The two principal methods that have been used to date have been transits and radial velocity. These techniques have yielded many hundreds of new detections and confirmed discoveries but are particularly sensitive to planets that are close to their host star.
While these detections are quite valuable to science, we have little information about the larger exterior exoplanets, analogs of our own Saturn and Caelus (Uranus). We have even less information about the numbers, spatial distribution and masses of free-floating planets - planets that are not gravitationally bound to any star. The most effective way to detect planets in distant orbits about their host star is through direct observation of stellar light reflected from the planet or using gravitational microlensing. Free-floating planets may only be detected using this last technique.
Why are these exterior and free-floating planets important to science?
Current, competing planet formation theories - mathematical models for how exoplanetary systems form and evolve - predict quite different numbers of exterior and free-floating planets. For example, recent refereed theoretical papers suggest that the frequency of free-floating planets could be as high as two times the number of stars in the Milky Way or as low as zero. Clearly, someone is wrong! These theoretical considerations need a statistically robust sample of free-floating planets to calibrate them, that is, to correct the models to the measurements, thereby making the theory - our human understanding of nature - closer to the underlying physical truth.
What is gravitational microlensing? What makes it different from other planet discovery techniques?
Microlensing uses the warping of spacetime to detect planets! As shown in the figure below, light from a distant star is emitted radially in the absence of any other mass. The observer, in this case, RST or CLEoPATRA, sees this distant star as a constant light source until an intervening mass comes between the source star and the observer. The intervening mass - in this case, a star with a planet - causes time to dilate or slow down near it.
Light always takes the path of least time and, as a result, the rays of light from the source star, which had been proceeding radially towards the observer, are briefly bent inwards. The observer sees this bending as a brightening then a dimming of the source star as the proper motion of the intervening mass carries it first into then out of the line of sight to the source star.
The brightening is caused by the magnification of the light from the source, with the quantity and duration of the magnification itself being the signature of the relative velocity, distance and quantity of the intervening mass. Importantly, this technique does not depend on the detection of light emission from the intervening body. It is sensitive to any massive object, from a free-floating planet to a neutron star or a black hole. Gravitational microlensing can even detect wormholes in spacetime if they exist! A more detailed and pedagogical description of gravitational microlensing may be found here.
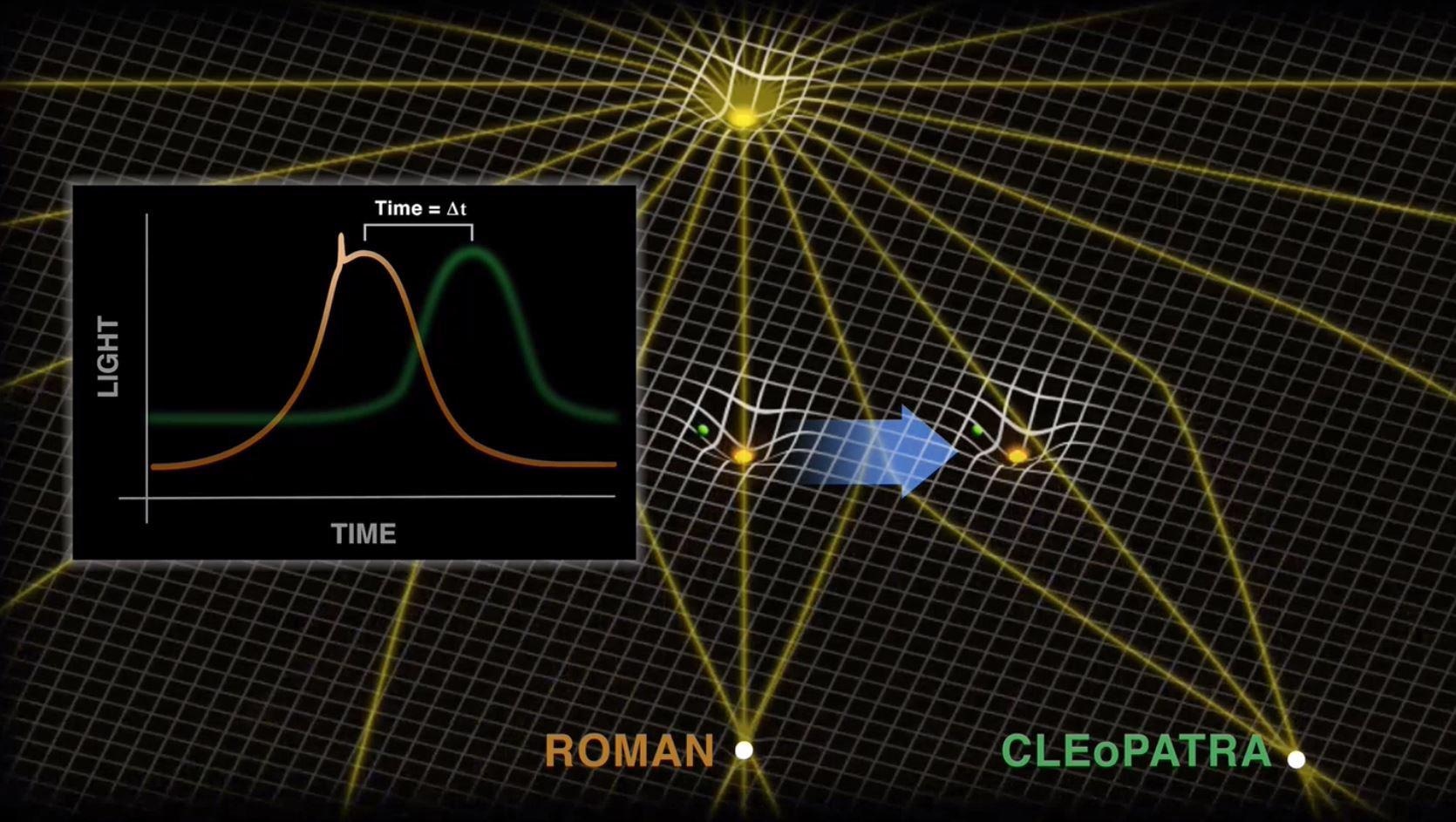
Image Credit: Dr. Richard K. Barry
What do you hope for the findings of CLEoPATRA's 2025 mission?
There are two parts of the CLEoPATRA mission — the cruise phase and the remote station phase. These phases have different scientific goals.
Shortly after the launch and commissioning of the observatory, during the cruise phase, CLEo will have two observational goals. First, she will observe the RST FOR to detect free-floating planets. Recent theory suggests that, while detectable at any baseline length given sufficient sensitivity, the masses of unbound planets may be determined by simultaneous measurement of microlensing parallax with a relatively short baseline of about 0.01 astronomical units or AU. (An AU is the average distance from Earth to the Sun.)
Subsequently, once the orbital baseline distance from RST at the second Earth-Sun Lagrangian point (or from terrestrial observatories) becomes too large to permit a mass estimate for free-floating planets, CLEo will observe a number of nearby red dwarf stars that are known to host exoplanets. In this phase, she will look specifically for flares and other evidence of chromospheric activity of these planet-hosting stars as such activity may significantly impact the onset and evolution of extraterrestrial life.
During the remote station phase of the mission, CLEoPATRA will provide a parallactic baseline length exceeding 0.2 AU or about 30 million kilometers. At this much longer separation, the time of the peak brightening of the microlensing event, relative to the time of peak brightening detected by RST or terrestrial observatories, will be used to estimate the distance to the microlensing system. This will permit a low-uncertainty measurement of the masses of the star and its planetary companion.
What information could CLEoPATRA provide regarding planetary formation?
Theory suggests that planetary systems form from the condensation of gas and dust in the interstellar medium. As the clump of material slowly collapses under the influence of its own gravity, the central region may reach the point at which its density causes hydrogen atoms to fuse into helium, releasing a vast amount of energy. The radiation produced by this process pushes the gas and dust outwards until the outward radiation pressure and the inward gravitational force reach equilibrium: A star is born.
The faint, diaphanous material around the new central star continues to contract and flatten into a slowly revolving disk. Local clustering of matter around existing, small primordial over-densities in the interstellar medium nucleate into planetesimals, and eventually, planets. As the mass of the system becomes more centrally concentrated, the forming exoplanetary system ‘spins up’ like a skater pulling his arms in while pirouetting. As the new system settles into a dynamically relaxed state some planetary orbits will circularize while others are pumped up - the total kinetic energy of the system being constant.
Some of these newly formed planets may gain enough energy in this process to be pushed into increasingly eccentric parabolic orbits. With sufficient energy input, the orbit of these planets may open - becoming hyperbolic - thus reaching escape velocity from the forming exoplanetary system altogether. These so-called ‘rogue’ planets carry away excess energy and angular momentum, thereby permitting planets in the system to settle into mostly circularized orbits, much as we see today in Earth’s own host stellar planetary system.
Current competing theories about this process predict different number densities of distantly bound planets and unbound, rogue planets. RST and CLEoPATRA will permit a direct measurement of numbers and masses of these bodies to facilitate calibration of theory.
Can you give an insight into CLEoPATRA's use of AI?
This is one of the really exciting parts of the CLEoPATRA mission! The motivation for the use of AI by the probe is primarily due to the great cost of communications between the spacecraft and Earth when CLEo reaches her remote station at a distance of about 0.2 AU. As the distance between these two bodies increases the amount of power dedicated to communications must increase apace - the absolute flux of the radio signals dropping as the square of the distance between transmitter and receiver. At large separations this energy cost can become a dominant term in the overall cost of a space mission.
Enter MAtISSE.
We have worked many years on a dependable mechanism to reduce the required data bandwidth for small spacecraft - with the CLEoPATRA mission in mind. Motivated by the need to communicate wide-field imaging information over great distances we have explored two primary techniques: compressive sensing and artificial intelligence. Compressive sensing is a method of using a sampling matrix to reduce the total volume of data as it is collected - changing a large NxN matrix of imaging information into an MxN representative vector.
The same sampling matrix may then be used on the ground to reconstruct the original data, using the inverse process, with very little loss. This technique is showing great promise and, though not quite ready for deployment on the mission, is the electrical engineering doctoral thesis work of one of CLEo’s graduate students.
While our compressive sensing research has remained vigorously active, we have made a great deal of headway on our AI umbrella project - MAtISSE: MAchine Intelligence for Small SatellitEs. This effort was funded through NASA’s Astrophysics grants program to develop and test our neural network and qualify low-power fanless GPUs, typically used for terrestrial industrial machines or aerial drones, for use in space. (To simulate the radiation environment of space, we use hospital proton accelerators ‘after hours’ when they are not being employed to treat cancers.)
While our calculations and experiments now show that inferencing using our neural net is sufficiently rapid for the current project using standard radiation-hardened CPUs, we continue to evaluate GPUs under space-like conditions anticipating a need for much faster inferencing for short-duration events such as microlensing associated with free-floating planets.
We have focused a great deal of effort over the past five years on the development of our neural network, RAMjET: RApid Machine lEarned Triage. Beginning as a simple Support Vector Machine using Time Warp Edit Distance for classification, we now employ a deep neural net that we are developing using NASA’s GPU supercomputer. Once the time-intensive training of the neural net is complete, actual inferencing is very rapid, taking only tens of milliseconds per lightcurve even on a CPU or low-power GPU. This inferencing will be conducted by CLEo, autonomously, in deep space!
There is a significant advantage to using AI to conduct classification and down-select of science data on the CLEoPATRA spacecraft rather than on the ground - especially for very rare events such as gravitational microlensing by planets.
CLEo’s instrument payload will image the dense star fields of the RST FOV in the Galactic bulge every ten minutes using a 4k x 4k detector array of 32-bit pixels resulting in single files exceeding 64 MB. Crucially, most of the stars in each image will be quiescent or will exhibit a kind of variability that is not of interest for the scientific goals of CLEoPATRA.
Consequently, downlinking these full-frame images would be scientifically unhelpful at best and prohibitively expensive at worst. Moreover, the time needed to downlink through NASA’s Deep Space Network would be a significant driver in the overall cost of the CLEoPATRA mission and would greatly reduce observing efficiency. Our pioneering solution for this problem, unique to CLEo, is to conduct a significant part of the pre-processing and inferencing on the spacecraft! To illustrate the savings in data bandwidth we describe the steps that CLEo conducts before downlink.
During the long-baseline, remote station phase of the mission, CLEoPATRA will image the Galactic bulge, as described above, resulting in the acquisition of large, full-frame images (FFI) every ten minutes. The first step in reducing the data is accomplished automatically by subtracting the new image from a co-registered reference image. The result differenced image is a sparse matrix that includes information only about objects that changed in brightness over the past ten minutes. The onboard computer tracks any object that changed, adding a measurement of the object’s brightness to a time-ordered list - called a lightcurve - stored in memory. Lightcurves, so accumulated, are presented to RAMjET to determine which type of stellar variability they exhibit.
Some examples of stellar variability that are not microlensing are pulsations, recurrent novae, supernovae, exoplanetary transits, flares, tidal distortions, binarity - to name a few. RAMjET identifies these and rejects all variables that do not exhibit microlensing. Finally, only those lightcurves that RAMjET assigns high likelihood of microlensing are accumulated in an onboard solid-state recorder for downlink through the DSN once each month.
Astronomers on the ground receive these lightcurves, and, using the recorded spacecraft pointing information, associate each microlensing event with the same event detected by RST. The difference between the time of peak magnification of the event as detected at each of RST and CLEoPATRA is noted, resulting in an estimate of a distance to the lensing object.
Importantly, CLEo may store neural nets for several different types of stellar phenomenology or could have a new neural net uplinked to refine her search criteria as new information becomes known or new scientific goals are identified. (Detection of stellar flare events during the cruise phase will require a different neural net for inferencing, for example. This will be stored onboard and loaded into the inferencing computer when needed by the mission.)
CLEoPATRA will essentially be an autonomous deep-space drone capable of searching for astronomical phenomena on her own, letting Earth-bound observers know when she has found something of interest!
The important advancement we describe here is to have significant intelligence on board to permit the efficient observation of rare astronomical events by a small, energy-starved, bandwidth-limited spacecraft. The modus operandi of most of NASA’s spacecraft - including the Roman Space Telescope - is to downlink whole FFI and to extract the actual scientific information after the fact.
This process requires so much energy and time that it is prohibitive for small spacecraft such as CLEo, especially spacecraft at a great distance from Earth. While the archived FFI from RST and other large observatories may be valuable for other archival science, purpose-built, intelligent, autonomous spacecraft, such as CLEoPATRA, have the potential to open vast new discovery spaces for science at very low cost.
Where can readers find more information?
Readers can find more information on my website.
About Dr. Richard K. Barry
Richard K. Barry is an American astronomer who works as a research scientist for NASA in the Laboratory for Exoplanets and Stellar Astrophysics. He is a former United States Air Force mechanic, NASA Space Shuttle Engineer and NASA electromechanical design engineer. Dr. Barry is the Observatory Project Scientist for the Hubble Space Telescope (HST) and holds three degrees in avionics and electrical engineering and two in physics and astronomy. His research includes exoplanet transits and microlensing and self-lensing black holes. Dr. Barry has twice won the Robert H. Goddard award for mentoring. He is a private pilot and enjoys scuba diving and competitive sailing. The image above was taken in the HST control room at NASA.
More information about Dr. Barry can be found here.
Disclaimer: The views expressed here are those of the interviewee and do not necessarily represent the views of AZoM.com Limited (T/A) AZoNetwork, the owner and operator of this website. This disclaimer forms part of the Terms and Conditions of use of this website.